
Firstname name
Function
I'm a paragraph. I'm connected to your collection through a dataset. To update me, go to the Data Manager. The Data Manager is where you store data to use in your site pages, or collect data from site visitors when they submit a form.
This collection in the Data Manager is already set up with some fields and content. To customize it with your own content, you can import a CSV file or simply edit the placeholder text. You can also add more fields which you can connect to other page elements so the content displays on your published site. Remember to sync the collection so your content is live! You can add as many new collections as you need to store or collect data.

Firstname name
Function
I'm a paragraph. I'm connected to your collection through a dataset. To update me, go to the Data Manager. The Data Manager is where you store data to use in your site pages, or collect data from site visitors when they submit a form.
This collection in the Data Manager is already set up with some fields and content. To customize it with your own content, you can import a CSV file or simply edit the placeholder text. You can also add more fields which you can connect to other page elements so the content displays on your published site. Remember to sync the collection so your content is live! You can add as many new collections as you need to store or collect data.

Firstname name
Function
I'm a paragraph. I'm connected to your collection through a dataset. To update me, go to the Data Manager. The Data Manager is where you store data to use in your site pages, or collect data from site visitors when they submit a form.
This collection in the Data Manager is already set up with some fields and content. To customize it with your own content, you can import a CSV file or simply edit the placeholder text. You can also add more fields which you can connect to other page elements so the content displays on your published site. Remember to sync the collection so your content is live! You can add as many new collections as you need to store or collect data.

Firstname name
Function
I'm a paragraph. I'm connected to your collection through a dataset. To update me, go to the Data Manager. The Data Manager is where you store data to use in your site pages, or collect data from site visitors when they submit a form.
This collection in the Data Manager is already set up with some fields and content. To customize it with your own content, you can import a CSV file or simply edit the placeholder text. You can also add more fields which you can connect to other page elements so the content displays on your published site. Remember to sync the collection so your content is live! You can add as many new collections as you need to store or collect data.

Firstname name
Function
I'm a paragraph. I'm connected to your collection through a dataset. To update me, go to the Data Manager. The Data Manager is where you store data to use in your site pages, or collect data from site visitors when they submit a form.
This collection in the Data Manager is already set up with some fields and content. To customize it with your own content, you can import a CSV file or simply edit the placeholder text. You can also add more fields which you can connect to other page elements so the content displays on your published site. Remember to sync the collection so your content is live! You can add as many new collections as you need to store or collect data.

Firstname name
Function
I'm a paragraph. I'm connected to your collection through a dataset. To update me, go to the Data Manager. The Data Manager is where you store data to use in your site pages, or collect data from site visitors when they submit a form.
This collection in the Data Manager is already set up with some fields and content. To customize it with your own content, you can import a CSV file or simply edit the placeholder text. You can also add more fields which you can connect to other page elements so the content displays on your published site. Remember to sync the collection so your content is live! You can add as many new collections as you need to store or collect data.

Firstname name
Function
I'm a paragraph. I'm connected to your collection through a dataset. To update me, go to the Data Manager. The Data Manager is where you store data to use in your site pages, or collect data from site visitors when they submit a form.
This collection in the Data Manager is already set up with some fields and content. To customize it with your own content, you can import a CSV file or simply edit the placeholder text. You can also add more fields which you can connect to other page elements so the content displays on your published site. Remember to sync the collection so your content is live! You can add as many new collections as you need to store or collect data.
Présentation
Lorsqu'elles sont attaquées par un pathogène, les plantes sont capables de mettre en place des mécanismes de défense qui vont ralentir plus ou moins efficacement, et parfois stopper complètement l'infection. Ces défenses inductibles comprennent, par exemple, la production de molécules ayant des propriétés antimicrobiennes, comme les molécules de la famille des glucosinolates et des isoflavones. Un mécanisme de défense des plantes appelé réponse hypersensible repose sur l'autodestruction rapide des cellules végétales bordant le point d'infection (Fig. 1).
Restez connecté et suivez-nous sur Twitter @QIPlab

Figure 1. Quelques symptômes de maladies végétales révélateurs des mécanismes moléculaires régissant l'interaction plante-pathogène. (A) Réaction hypersensible typique (cellules mortes entourées d'un halo jaunâtre) sur une feuille de A. thaliana conduisant au confinement des bactéries pathogènes dans une zone limitée de la feuille, puis à leur destruction. (B) Jeune plant de tournesol infecté par l'oomycète du mildiou Plasmopara halstedii, formant des sporanges blancs à la surface. Les tissus infectés restent vivants et verts en réponse à ce pathogène biotrophe. (C) Plante de tournesol infectée par le champignon de la pourriture blanche Sclerotinia sclerotiorum. Les organes de la plante infectée meurent rapidement, produisant des lésions brunâtres caractéristiques d'une interaction nécrotrophe.
En l'absence de résistance qualitative, on observe souvent un phénotype de résistance partielle ou incomplète, se traduisant par une réduction des symptômes plutôt que par une absence de maladie sur une plante donnée. Au niveau des populations végétales, ce phénomène se traduit par une distribution continue des niveaux de résistance et est appelé résistance quantitative aux maladies (QDR, Fig. 2). L'émergence d'un phénotype de résistance qualitative ou quantitative dépend du génotype de l'agent pathogène et du génotype de la plante, selon le modèle de verrouillage moléculaire mentionné ci-dessus, mais aussi des conditions environnementales (conditions pédoclimatiques et autres microorganismes présents). Ainsi, comprendre la nature et la régulation des processus biologiques qui contribuent au phénotype de résistance quantitative est essentiel pour optimiser la durabilité de la résistance des plantes face au pouvoir évolutif des populations de pathogènes et aux variations des conditions environnementales.
L'architecture génétique du caractère de résistance étudié est souvent riche en informations sur les contraintes évolutives et fonctionnelles qui pèsent sur l'immunité des plantes, mais elle est parfois difficile à approcher. La cartographie des loci de résistance quantitative conduit généralement à l'identification de multiples loci de caractères quantitatifs (QTL) d'effet faible à modéré, expliquant entre 5 et 30% de la variance phénotypique. En théorie, la résistance qualitative se distingue donc par la possibilité d'obtenir une résistance complète par l'introduction d'un seul gène (dit de "résistance" ou "R") dans un fond génétique sensible, alors que l'introduction d'un gène de résistance quantitative ne conférera qu'une résistance partielle. En pratique, cependant, certains gènes R peuvent ne conférer qu'une résistance partielle selon l'allèle considéré, les conditions environnementales ou le génotype du pathogène étudié (Fig. 2). Inversement, un phénotype de résistance quantitative peut apparaître binaire (résistant/sensible typique de la résistance qualitative) si la population végétale étudiée manque de diversité, si les conditions environnementales gomment les différences phénotypiques, ou si la précision et la résolution de la méthodologie de phénotypage sont insuffisantes. La caractérisation fonctionnelle des QTL de résistance nécessite donc des technologies robustes et précises pour (i) cartographier les loci de résistance quantitative sur le génome de la plante et (ii) évaluer la contribution phénotypique d'un gène ou d'un allèle au locus de résistance.
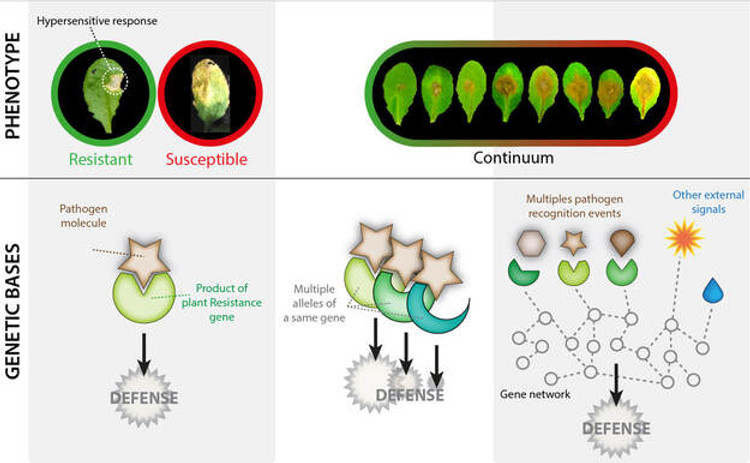
Figure 2. Différentes formes de résistance des plantes. Elles sont caractérisées par la distribution du phénotype de résistance au sein d'une population hôte (binaire ou continuum de résistance), ainsi que par l'architecture des bases génétiques impliquées. Les molécules produites par l'agent pathogène sont représentées en brun, les molécules produites par la plante sont représentées en vert. Dans le réseau de gènes, chaque cercle représente un gène de la plante. Les lignes pointillées représentent les interactions entre les éléments du réseau. Les "autres signaux externes" correspondent à des signaux de l'environnement indépendants du pathogène, tels que l'intensité lumineuse, l'humidité, etc. Les "autres signaux externes" correspondent aux signaux de l'environnement indépendants de l'agent pathogène, tels que l'intensité lumineuse, l'humidité, etc.
Lecture recommandée :
-
Genome evolution in filamentous plant pathogens: why bigger can be better. www.nature.com/articles/nrmicro2790
-
Resistance to phytopathogens e tutti quanti: placing plant quantitative disease resistance on the map. bsppjournals.onlinelibrary.wiley.com/doi/full/10.1111/mpp.12138
-
Advances on plant-pathogen interactions from molecular toward systems biology perspectives. onlinelibrary.wiley.com/doi/full/10.1111/tpj.13429
-
Silent control: microbial plant pathogens evade host immunity without coding sequence changes. academic.oup.com/femsre/advance-article/doi/10.1093/femsre/fuab002/6095737
Sclerotinia, la moisissure qui frappe fort
Le pathogène de la moisissure blanche et de la moisissure de la tige, Sclerotinia sclerotiorum, est un champignon pathogène généraliste, qui infecte une large gamme d'espèces hôtes (>400) dans la nature. Son nom vient du grec ancien σκληρός (sklērós) qui signifie "dur", en référence aux structures de repos dures et mélanisées appelées sclérotes qu'il forme. Ce mot grec signifie également "dur", ce qui reflète bien le comportement des Sclerotinia avec les plantes. Il fait partie des agents pathogènes des plantes les plus dévastateurs au monde et provoque des maladies sur de nombreuses cultures, notamment le soja, le colza, le tournesol et la plupart des légumes. Les solutions pour le contrôle génétique de la maladie sont très limitées dans la plupart des cultures. S. Sclerotiorum infecte naturellement les espèces sauvages et cultivées de Brassica, y compris la plante modèle Arabidopsis thaliana qui présente une résistance quantitative à la maladie lorsqu'elle est attaquée par Sclerotinia.
Ces dernières années, la majorité des études sur les interactions entre les plantes et les champignons pathogènes se sont concentrées sur des interactions hôte-pathogène étroitement couplées. Par exemple, le champignon de l'oïdium Blumeria graminis, les champignons de la rouille des céréales Puccinia spp. et le champignon du charbon du maïs Ustilago maydis sont parmi les champignons pathogènes les plus étudiés et sont des pathogènes biotrophes obligatoires limités à un seul genre d'hôte. Ces interactions par paires ne représentent qu'une fraction des interactions plantes-fongiques pathogènes rencontrées dans la nature et un certain nombre de champignons pathogènes à large gamme d'hôtes constituent également des menaces majeures pour la sécurité alimentaire. Comprendre comment les agents pathogènes à large gamme d'hôtes réussissent à infecter plusieurs lignées de plantes est un défi majeur en phytopathologie.
Parmi les Léotiomycètes, le champignon de la moisissure grise Botrytis cinerea et le champignon de la moisissure blanche Sclerotinia sclerotiorum se distinguent par une gamme d'hôtes remarquablement large, comprenant plus de 200 espèces. Chacun de ces agents pathogènes provoque chaque année des pertes de récoltes de plusieurs centaines de millions de dollars US dans le monde. Ils sont considérés comme des nécrotrophes typiques, sécrétant un arsenal d'enzymes et de toxines dégradant la paroi cellulaire pour tuer les cellules hôtes et en tirer de l'énergie. Les plantes hôtes présentent généralement une résistance quantitative aux maladies (QDR) à B. cinerea et S. sclerotiorum, ce qui entraîne une réduction plutôt qu'une absence de la maladie. La manière dont ces champignons pathogènes généralistes provoquent des maladies et les bases génétiques de la résistance quantitative des plantes sont encore mal comprises et constituent un axe majeur de nos recherches.

Figure 3. The plant pathogenic fungus Sclerotinia sclerotiorum.
When attacked by a pathogen, plants are able to set up defense mechanisms that will slow down more or less effectively, and sometimes stop the infection completely. These inducible defenses include, for example, the production of molecules with antimicrobial properties, such as molecules of the glucosinolate and isoflavone family. A plant defense mechanism called hypersensitive response relies on the rapid self-destruction of plant cells bordering the point of infection (Fig. 1). By radically modifying the pathogen's environment, the hypersensitive response can completely inhibit the infection and stop the disease completely. This is called complete resistance or qualitative resistance. The hypersensitive response is particularly effective against biotrophic pathogens, which can only complete their infectious cycle in a living host (Fig. 1). Moreover, the triggering of the hypersensitive response requires the recognition of specific molecules of certain pathogen genotypes by dedicated plant receptor proteins. This form of defence is thus controlled by a more or less elaborate key-lock mechanism that is reconstituted only when plant-pathogen pairs with compatible keys and locks are present (Fig. 2). Varietal selection is often aimed at favouring plants capable of recognising genotypes of pathogens common in agricultural environments. Minor variations in the "molecular key" carried by the pathogen may nevertheless render the plant detection system inoperative (pathogen evading resistance). On the other hand, many pathogens are able to complete their infectious cycle in dead host tissue, and necrotrophic agents will even actively induce host cell death to complete their cycle (Fig. 1). Consequently, the hypersensitive response and the resulting complete resistance remain exceptional phenomena in nature.
Figure 1: Some plant disease symptoms indicative of the molecular mechanisms governing plant-pathogen interaction. (A) Typical hypersensitive reaction (dead cells surrounded by a yellowish halo) on a leaf of A. thaliana leading to the confinement of pathogenic bacteria in a limited area of the leaf, then to their destruction. (B) Young sunflower seedling infected with the downy mildew oomycete Plasmopara halstedii, forming white sporangia on the surface. Infected tissues remain alive and green in response to this biotrophic pathogen. (C) Sunflower plant infected with the white rot fungus Sclerotinia sclerotiorum. The infected plant organs die rapidly, producing brownish lesions characteristic of a necrotrophic interaction
In the absence of qualitative resistance, a phenotype of partial or incomplete resistance is often observed, resulting in a reduction of symptoms rather than absence of disease on a given plant. At the level of plant populations, this phenomenon results in a continuous distribution of resistance levels and is referred to as quantitative disease resistance (QDR, Fig. 2). The emergence of a qualitative or quantitative resistance phenotype depends on the genotype of the pathogen and the plant genotype, according to the molecular key lock model mentioned above, but also on environmental conditions (soil and climate conditions and other microorganisms present). Thus, understanding the nature and regulation of the biological processes that contribute to the quantitative resistance phenotype is essential for optimizing the durability of plant resistance in the face of the evolutionary power of pathogen populations and variations in environmental conditions.
The genetic architecture of the resistance trait studied is often rich in information on the evolutionary and functional constraints that weigh on plant immunity, but is sometimes difficult to approach. The mapping of quantitative resistance loci typically leads to the identification of multiple quantitative trait loci (QTLs) of low to moderate effect, explaining between 5 and 30% of the phenotypic variance. In theory, qualitative resistance is therefore distinguished by the possibility of obtaining complete resistance by the introduction of a single gene (known as "resistance" or "R" gene) into a sensitive genetic background, whereas the introduction of a quantitative resistance gene will only confer partial resistance. In practice, however, some R genes may only confer partial resistance depending on the allele considered, the environmental conditions or the genotype of the pathogen under study (Fig. 2). Conversely, a quantitative resistance phenotype may appear binary (resistant/sensitive typical of qualitative resistance) if the plant population under study lacks diversity, if environmental conditions erase phenotypic differences, or if the precision and resolution of the phenotyping methodology is insufficient. Functional characterization of resistance QTLs therefore requires robust and accurate technologies to (i) map quantitative resistance loci on the plant genome and (ii) assess the phenotypic contribution of a gene or allele to the resistance locus.
Figure 2. Different forms of plant resistance. These are characterized by the distribution of the resistance phenotype within a host population (resistance-sensitive binary or continuum), as well as by the architecture of the genetic bases involved. Molecules produced by the pathogen are shown in brown, molecules produced by the plant are shown in green. In the gene network, each circle represents a gene of the plant. The dotted lines represent interactions between the elements of the network. The "other external signals" correspond to signals from the environment that are independent of the pathogen, such as light intensity, humidity, etc. The "other external signals" correspond to signals from the environment that are independent of the pathogen, such as light intensity, humidity, etc.
Recommended reading:
-
Genome evolution in filamentous plant pathogens: why bigger can be better. www.nature.com/articles/nrmicro2790
-
Resistance to phytopathogens e tutti quanti: placing plant quantitative disease resistance on the map. bsppjournals.onlinelibrary.wiley.com/doi/full/10.1111/mpp.12138
-
Advances on plant-pathogen interactions from molecular toward systems biology perspectives. onlinelibrary.wiley.com/doi/full/10.1111/tpj.13429
-
Silent control: microbial plant pathogens evade host immunity without coding sequence changes. academic.oup.com/femsre/advance-article/doi/10.1093/femsre/fuab002/6095737
Sclerotinia, the hard-hitting mold
The white and stem mold pathogen Sclerotinia sclerotiorum is a generalist fungal pathogen, infecting a broad range of host species (>400) in nature. Its name comes from the from Ancient Greek σκληρός (sklērós) which means “hard", by reference to the hard and melanized resting structures called sclerotia that it forms. This greek word also means "tough”, which accurately reflects how Sclerotinia behave with plants. It is among the most devastating plant pathogens worldwide and causes disease on many crops including soybean, rapeseed, sunflower and most vegetables. Solutions for the genetic control of the disease are very limited in most crops. S. Sclerotiorum naturally infects wild and cultivated Brassica species, including the model plant Arabidopsis thaliana which shows quantitative disease resistance when challenged by Sclerotinia.
A majority of studies on plant interactions with fungal pathogens over the last years have focused on tightly coupled host-pathogen interactions. For instance the powdery mildew fungus Blumeria graminis, the cereal rust fungi of the Puccinia spp., and the corn smut fungus Ustilago maydis are among the most studied fungal pathogens and are obligate biotrophic pathogens restricted to a single host genus. Such pairwise interactions only represents a fraction of plant-fungal pathogen interactions encountered in nature and a number of broad host range fungal pathogens also are major threats for food security. Understanding how broad host range pathogens successfully infect multiple plant lineages is a major challenge in plant pathology.
Among Leotiomycete, the grey mold fungus Botrytis cinerea and the white mold fungus Sclerotinia sclerotiorum stand out for having a remarkably broad host range, encompassing over 200 species. Each of these pathogens causes yearly several hundred millions of US dollars crop losses worldwide. They are considered as typical necrotrophs, secreting an arsenal of cell wall-degrading enzymes and toxins to kill host cells and derive energy. Host plants typically exhibit quantitative disease resistance (QDR) to B. cinerea and S. sclerotiorum, leading to a reduction rather than absence of disease. How these generalist fungal pathogens cause disease and what are the genetic bases of plant QDR is still poorly understood and a major focus of our research.
Figure 3. The plant pathogenic fungus Sclerotinia sclerotiorum.
Recommended reading:
-
Emerging Trends in Molecular Interactions between Plants and the Broad Host Range Fungal Pathogens Botrytis cinerea and Sclerotinia sclerotiorum. www.frontiersin.org/articles/10.3389/fpls.2016.00422/full
-
Shifts in diversification rates and host jump frequencies shaped the diversity of host range among Sclerotiniaceae fungal plant pathogens. onlinelibrary.wiley.com/doi/full/10.1111/mec.14523
-
Codon optimization underpins generalist parasitism in fungi. elifesciences.org/articles/22472
-
Intercellular cooperation in a fungal plant pathogen facilitates host colonization. www.pnas.org/content/116/8/3193.long
RESEARCH THEMES

Légende de la figure : A) Gamme des symptômes sur des feuilles d’Arabidopsis thaliana inoculées par S. sclerotiorum avec une illustration de l’ « image processing » utilisé pour quantifier la. B) Structure moléculaire de la proteine fongique predate pour faciliter la colonisation de l’hôte. C) Genome wide association mapping de gènes végataux contribuant à la QDR, avec une carte du monde indiqant l’origine des accessions de plantes utilisées
Publications récentes représentatives :
Bozkurt TO, Richardson A, Dagdas YF, Mongrand S, Kamoun S, Raffaele S. The Plant Membrane-Associated REMORIN1.3 Accumulates in Discrete Perihaustorial Domains and Enhances Susceptibility to Phytophthora infestans. Plant Physiol. 2014 May 7;165(3):1005-1018.
Contact : Sylvain Raffaele
Caracterisation des mécanismes moléculaires sous-tendant l’immunité quantitative chez Arabidopsis au champignon Sclerotinia sclerotiorum
Grâce à l’utilisation de populations mondiales d’Arabidopsis thaliana, nous avons mis en évidence une forte variation de l’immunité quantitative à S. sclerotiorum, ouvrant ainsi la voie à la caractérisation moléculaire des déterminants végétaux et fongiques de cette interaction. Les objectifs de ce projet sont d’identifier quels gènes végétaux sont impliqués dans l’immunité quantitative à S. sclerotiorum et de comprendre comment ils contribuent à la résistance. Pour cela, nous développons notamment des approches de genome wide association mapping, de génétique.
Thigmoimmunité: contribution du signal mécanique à l’immunité quantitative contre Sclerotinia
Durant leur interaction avec les plantes, et avant pénétration tissulaire et and dégradation, les pathogènes fongiques dévelopent des charges importantes mécaniques susceptibles d’émettre des signaux mécaniques (MS) et d’initier la défense des plantes. Les charges mécaniques sont dues à la forte pression de turgescence créée par l’eau de la vacuole des appressoria les propriétés mécaniques de la paroi fongique.
Ce stress mécanique est en général suffisant pour pénétrer dans les cellules végétales. Le “mechanosensing” intervient au niveau de la cellule végétale et repose sur l’état mécanique de la cellule.
Des études récentes démontrent un lien entre mechanosensing et réponse immunitaire de la plante à B. cinerea chez Arabidopsis thaliana: les plantes soumises au MS montrent une résistance accrue à l’infection fongique suggérant un effet de priming provoqué par un mechanosensing stérile. A l’avenir, nous essaierons de comprendre si le mechanosensing par contact fongique ou pénétration per se, en plus de la perception des PAMP, conduit à une résistance accrue de la plante.
Liens :
http://journal.frontiersin.org/article/10.3389/fpls.2016.00422/full
Mechanoperception and thigmomorphogenesis at INRA
http://www6.ara.inra.fr/piaf_eng/About/Teams/MECA
Publications récentes représentatives :
Mbengue M, Navaud O, Peyraud R, Barascud M, Badet T, Vincent R, Barbacci A, Raffaele S. Emerging Trends in Molecular Interactions between Plants and the Broad Host Range Fungal Pathogens Botrytis cinerea and Sclerotinia sclerotiorum. Front Plant Sci. 2016 Mar 31;7:422.
Barbacci A, Magnenet V, Lahaye M. Thermodynamical journey in plant biology. Front Plant Sci. 2015 Jun 30;6:481.
Contact : Adelin Barbacci
Adaptations fongiques à l’immunité quantitative végétale
Les champignons pathogènes constituent des menaces importantes pour l’alimentation et l’environnement. Les pathogènes fongiques généralistes comme S. sclerotiorum, qui infectent une large gamme d’espèces végétales dans la nature, sont parmi les plus dévastateurs au monde.
La gamme d’hôtes qu’un pathogène peut infecter dans la nature est un déterminant clé de l’émergence et de la propagation des maladies. Comment les pathogènes évoluent ils pour développer la capacité à infecter plusieurs hôtes, demeure une énigme. A travers ce projet, nous posons les questions suivantes: Quels sont les mécanismes utilises par Sclerotinia pour coloniser ses hôtes? Quels sont les processus évolutifs qui conduisent aux processus de virulence et d’immunité? Ce travail repose essentiellement sur des approches de génomique comparative, de transcriptomique, de phylogénie, et de biologie des systèmes.

Légende de la figure : A) S. sclerotiorum colonisant une feuille d’Arabidopsis en utilisant une souche exprimant la protéine GFP. B) Un diagramme Circos illustrant la génomique comparative des espèces fongiques relatives à S. sclerotiorum. C) Arbre phylogénétique des champignons de la famille des Sclerotiniaceae..
Publications récentes représentatives :
Dong S, Raffaele S, Kamoun S. The two-speed genomes of filamentous pathogens: waltz with plants. Curr Opin Genet Dev. 2015 Dec;35:57-65. doi: 10.1016/j.gde.2015.09.001.
Badet T, Peyraud R, Raffaele S. Common protein sequence signatures associate with Sclerotinia borealis lifestyle and secretion in fungal pathogens of the Sclerotiniaceae. Front Plant Sci. 2015 Sep 24;6:776.
Guyon K, Balagué C, Roby D, Raffaele S. Secretome analysis reveals effector candidates associated with broad host range necrotrophy in the fungal plant pathogen Sclerotinia sclerotiorum. BMC Genomics. 2014 May 4;15:336.
Contact : Sylvain Raffaele
Collaborations
-
Bruno Grezes-Besset; Biogemma Mondonville
-
Fabrice Roux; LIPM Toulouse
-
Sébastien Mongrand; LBM Bordeaux
-
John P. Clarkson; University of Warwick (UK)
-
Richard P. Oliver, Mark Derbyshire, Matthew Denton-Giles; Curtin University (Aus)
-
Lone Buschwaldt; University of Saskatchewan (Can)
-
Jan van Kan, Michael Siedl; Wageningen University (NL)
-
Sophien Kamoun; The Sainsbury Laboratory Norwich (UK)
-
Jean-Charles Portais, Pierre Millard; LISBP Toulouse
-
Ludovic Cottret, Lucas Marmiesse; LIPM Toulouse
Financements
-
Modulating the gene networks underlying phenotypic robustness in plant quantitative immunity (PROBITY).
Coordinator: Sylvain Raffaele - 442k€
-
ANR Projet "Level-UP"
-
ANR IProjet "ResiDEvo"
-
Metaprogramme Digitbio
MEMBERS
MEMBERS
2021
Kusch S, Larrouy J, Ibrahim HMM, Mounichetty S, Gasset N, Navaud O, Mbengue M, Zanchetta C, Lopez-Roques C, Donnadieu C, Godiard L, Raffaele S. Transcriptional response to host chemical cues underpins the expansion of host range in a fungal plant pathogen lineage. ISME J. 2021 Jul 19. doi: 10.1038/s41396-021-01058-x.
https://www.nature.com/articles/s41396-021-01058-x
Behind the paper: How do some pathogens infect hundreds of plants?
Ghosh R, Barbacci A, Leblanc-Fournier N. Mechanostimulation: a promising alternative for sustainable agriculture practices. J Exp Bot. 2021 Apr 2;72(8):2877-2888. doi: 10.1093/jxb/erab036.
academic.oup.com/jxb/article/72/8/2877/6123824
Chen H, Raffaele S, Dong S. Silent control: microbial plant pathogens evade host immunity without coding sequence changes. FEMS Microbiol Rev. 2021 Jan 13:fuab002. doi: 10.1093/femsre/fuab002.
academic.oup.com/femsre/advance-article/doi/10.1093/femsre/fuab002/6095737
Ibrahim HMM, Kusch S, Didelon M, Raffaele S. Genome-wide alternative splicing profiling in the fungal plant pathogen Sclerotinia sclerotiorum during the colonization of diverse host families. Mol Plant Pathol. 2021 Jan;22(1):31-47. doi: 10.1111/mpp.13006.
https://bsppjournals.onlinelibrary.wiley.com/doi/10.1111/mpp.13006
2020
Didelon M, Khafif M, Godiard L, Barbacci A, Raffaele S. Patterns of sequence and expression diversification associate members of the PADRE gene family with response to fungal pathogens. Front Genet. 2020 May 29;11:491. doi: 10.3389/fgene.2020.00491.
https://www.frontiersin.org/articles/10.3389/fgene.2020.00491/full
Kusch S, Ibrahim HMM, Zanchetta C, Lopez-Roques C, Donnadieu C, Raffaele S. A chromosome-scale genome assembly resource for Myriosclerotinia sulcatula infecting sedge grass (Carex sp.). Mol Plant Microbe Interact. 2020 Apr 20. doi:10.1094/MPMI-03-20-0060-A.
https://apsjournals.apsnet.org/doi/abs/10.1094/MPMI-03-20-0060-A
Sucher J, Mbengue M, Dresen A, Barascud M, Didelon M, Barbacci A, Raffaele S. Phylotranscriptomics of the Pentapetalae Reveals Frequent Regulatory Variation in Plant Local Responses to the Fungal Pathogen Sclerotinia sclerotiorum. Plant Cell. 2020 Apr 7. pii: tpc.00806.2019. doi: 10.1105/tpc.19.00806.
www.plantcell.org/content/early/2020/04/07/tpc.19.00806.long
Featured in: Carella P. All Together Now: Phylotranscriptomics Reveals Core Responses to Fungal Infection Across the Pentapetalae. Plant Cell. 2020
www.plantcell.org/content/early/2020/04/20/tpc.20.00298
Barbacci A, Navaud O, Mbengue M, Barascud M, Godiard L, Khafif M, Lacaze A, Raffaele S. Rapid identification of an Arabidopsis NLR gene as a candidate
conferring susceptibility to Sclerotinia sclerotiorum using time-resolved automated phenotyping. Plant J. 2020 Mar 14. doi: 10.1111/tpj.14747.
onlinelibrary.wiley.com/doi/abs/10.1111/tpj.14747